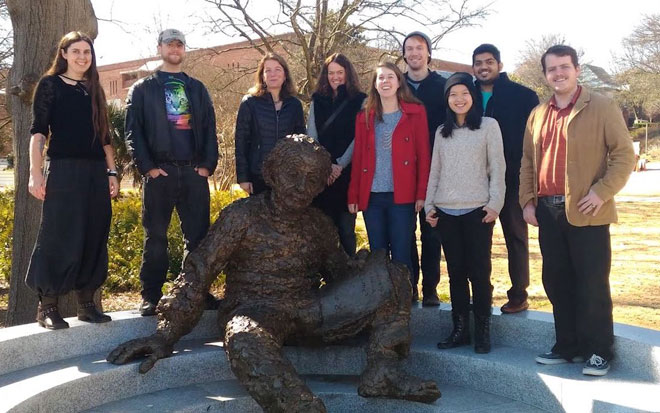
It took nearly 1.5 billion years to arrive. It was here for less than two hundred milliseconds. And its presence moved a pair of 2.5-mile vacuum tubes a distance of 1/400th the diameter of a proton. Yet despite its incredibly short stay and the microscopic movement, it is enough for scientists to claim one of the most significant discoveries in the world of physics this century.
For the first time ever, a gravitational wave has been observed. A team of global researchers announced the finding on Thursday, February 11. The discovery comes 100 years after Albert Einstein predicted the existence of gravitational waves in his theory of general relativity.
Gravitational waves are ripples in the very same fabric of the universe that bend and distort space-time. They are produced during violent cosmic disturbances.
In this case, the observed wave was created when two black holes collided approximately a billion and a half years ago, sending a ripple hurtling through space at the speed of light. It arrived on September 14, 2015, and was detected by LIGO – the Laser Interferometer Gravitational-Wave Observatory — a National Science Foundation-funded physics experiment that has searched for waves for more than a decade.
The LIGO Scientific Collaboration includes two Georgia Tech College of Sciences faculty members, and their team of 10 postdoctoral fellows, graduate, and undergraduate students. One of them is School of Physics Associate Professor Laura Cadonati, who chairs LIGO’s Data Analysis Council.
In this edition of Tech+Knowledge+Y, Cadonati explains how the waves were observed and why they unlock more secrets of the universe. The confirmation of Einstein’s prediction opens a new window of the cosmos, one that will provide humanity with better clues of how the universe was created and continues to evolve.
Expectations, and Career Goals, Confirmed
Relief. That’s what Deirdre Shoemaker says she felt when she saw proof of the gravitational wave last fall. After all, the director of Georgia Tech’s Center for Relativistic Astrophysics had planned her entire research career around the discovery, churning out hundreds of computer simulations for something that no one could guarantee actually existed. Shoemaker, a member of the LIGO Scientific Collaboration, solves Einstein’s equations for the collision of two binary black holes. All she needed was a real gravitational wave to see if her predictions were true.
“Gravitational waves are a vibration of space-time propagating toward us. To say it unscientifically, it’s like banging my fist on a table — you would feel a vibration if you were holding on to the other end. Any non-uniformly accelerating mass causes a gravitational wave — even moving your arms back and forth. But only very compact objects moving rapidly have a chance of being detected.
The signal from LIGO caught a lot of people by surprise. Most people thought the first one detected would be from a binary neutron star (a pair of the most compact stars known to exist). But this signal was unmistakable. It was from two relatively large black holes, something we didn't expect based on our astrophysical understanding. LIGO knew within minutes that we had something big. And I couldn’t have been more excited.
I don’t study neutron stars. I study binary black holes. So when I saw the signal, I knew something that strong could only be from colliding black holes. Our Georgia Tech team played a direct and pivotal role in the analysis of the observed signal. When the wave was detected at LIGO, it was a combination of the actual signal and background noise. Once that signal was extracted, our team was able to compare it with hundreds of our simulations of binary black hole mergers. This helped us confirm that the signal indeed originated from two black holes, nearly equal in mass, that were spinning on their axis as they orbited and collided, forming a single, spinning black hole.
We also created a visualization (seen further down this page) of the collision. The colors represent the ripples in space-time created by the black hole merger. It’s actually what happened 1.5 billion years ago. It’s 20 years of research and two weeks of dedicated time on a supercomputer solving Einstein’s equations."
VIDEO: Why Are Gravitational Waves So Important? Georgia Tech’s faculty and students played a role in the discovery and explain its historic significance.
Another Piece of the Universal Puzzle
A day like this was just a dream when Pablo Laguna started his doctoral work in the early 1980s. A science driven by gravitational wave observations is now a reality for the chair of the School of Physics. It’s one of three pillars thatsupport Georgia Tech’s Center for Relativistic Astrophysics (CRA), which he established in 2008.
“Gravitational waves, particle astrophysics, and high-energy astrophysics. These are the three pillars around which the CRA has assembled a research team comprising multi-messenger astrophysicists. It’s one of the fastest-growing astrophysical centers in the nation.
Why are we devoted to multi-messenger astrophysics? There are only three types of messengers that carry information about the cosmos: photons, or light, particles like neutrinos or cosmic rays and gravitational waves. Only centers like the CRA are uniquely positioned to enable research that cuts across these channels.
As LIGO’s observations become routine, our group and others will learn more about the populations of black holes and neutron stars. Those findings will provide us additional information about the lives of the stars that, after their death, leave behind black holes and neutron stars. In turn, these findings will shed light on the birth of the stars and the environments where they form, thus completing the life cycle of the universe.
LIGO’s detected wave, officially deemed GW150914, has given us the first snapshot of one of the most violent events in the universe — the collision of two black holes. It’s an essential piece of the puzzle that is our universe, a piece that allows my colleagues and I not only to test if Einstein was right, but also to investigate astrophysical phenomena where gravity has the strongest grip."
VIDEO:
When Black Holes Collide. A binary by Georgia Tech’s team including Matt Kinsey, Karan Jani and Michael Clark. (No audio.)
Reactions from Other Astrophysicists
As Laguna noted, gravitational waves form just one of the pillars of the Center for Relativistic Astrophysics.David Ballantyne and Tamara Bogdanovic depend on light to study the universe, particularly focusing on black holes, galaxies, and stars. Instead of light, Ignacio Taboada observes particles: cosmic rays, neutrinos, and gamma rays.
Taboada:
Until recently, and since the dawn of time, light had been humanity’s only astronomical messenger. Then researchers discovered neutrinos, which can be studied in particularly dense regions where light cannot escape. That’s because neutrinos are ghost-like: they can go through almost everything without being stopped. I’m a member of the IceCube collaboration, which has discovered astrophysical neutrinos that likely originated far away from our galaxy. Just like neutrinos, gravitational waves will allow us to peek deep into very violent phenomena where no light is emitted.
Bogdanovic:
Scientists studying gravitational waves are concerned about the waves black holes create as they draw closer to each other and collide. Astrophysicists like me fill in the spaces: where did the black holes come from, what pulled them together, and the kinds of galaxies they live in.
Ballantyne:
Our field is often referred to as “traditional astronomy,” which is based on the astronomical techniques of the last 100 years or so. I focus on the interaction of radiation produced by growing black holes with gas and dust clouds in galaxies. We use all of this information to figure out the physical processes occurring around black holes and their role in the evolution of their host galaxies. Studying gravitational waves is examining the basic laws of the universe — it’s like looking at the notes of the symphony. Tamara and I try to understand how that symphony affects the audience.
Bogdanovic:
That’s right. Gravitational waves tell us about the collision of two black holes. You learn about everything else around them by looking at the light emitted by the surrounding gas and stars in the host galaxy. When you put both light and gravitational waves together, you can place the merging black holes in a broader context of the universe.
Ballantyne:
General relativity has been a good description of gravity — it has passed every test people have thrown at it. Although I’m not surprised that they’ve been detected, I’m excited because gravitational waves open a new window of the universe.
Bogdanovic:
Each time one of these windows opens, it presents a brand new picture of the universe. Gravitational waves will inevitably bring confirmations of some previous expectations, as well as some surprises that will lead to new discoveries. I’m glad that it’s happening during my professional career because these types of developments don’t come around very often.
VIDEO: A binary black hole merger visualization by SXS.
Refining Scientific Ideas Adds More Than Polish
Georgia Tech College of Sciences Dean Paul Goldbart is proud of his colleagues and the work they’ve done in the months and years leading to the announcement of gravitational waves. He’s the former chair of the School of Physics and calls the new findings awe-inspiring.
"Why the excitement about gravitational waves? Refining scientific ideas adds more than polish. It can bring us major advances, too. Think of a pair of electric charges exerting forces on one another. That’s Coulomb’s law, an example of action-at-a-distance, with like charges repelling and opposing ones attracting. Faraday’s field idea moved us to a new viewpoint in which charges cause electric fields that permeate space — stronger fields near them and weaker ones farther away — with the electric fields pushing charges. Now for the refinement. The field isn’t just a device to hide action-at-a-distance. It has its own mechanical reality, as we’ll see shortly. What about magnetic fields? They’re also caused by charge — not its presence but its motion (Ampère’s law) — and when magnetic fields change in time they cause electric fields (Faraday again).
Here’s where modern physics begins. Just over 150 years ago, reflecting on what was known about electricity and magnetism, James Clerk Maxwell divined a new piece of the puzzle: If changing magnetic fields cause electric fields, perhaps changing electric fields cause magnetic fields? They do! And with this masterstroke, Maxwell uncovered the nature of light: Changing electric fields cause changing magnetic fields cause changing electric fields … in self-sustaining patterns, launched but not maintained by moving charges, that run through space at a speed that Maxwell’s theory settles: the speed of light. Note the progression: action-at-a-distance, fields, electromagnetic radiation. And by surveying the sky at different wavelengths of this radiation — gamma rays, X-rays, light, microwave, radio — these messengers have brought us rich, detailed pictures of the cosmos and its astrophysical inhabitants, ever since the Big Bang.
Now ask what happens if we replace charge by mass. Newton taught us about the force called gravity, which acts between masses — action-at-a-distance again — and in the late 1700s, Laplace introduced the gravitational field concept. Then, in a dramatic refinement motivated by the equality of mass in inertia and gravity, Einstein conjured up his General Theory of Relativity, which holds that mass (and energy) cause space (and time) to warp, and that this warping has its own dynamical reality: Space and time are no longer stage, but actor, it’s sometimes said. And 100 years ago this year, Einstein could echo Maxwell and foresee the possibility of new waves — gravitational radiation shone out into the cosmos as wisp-like ripples not in but of space and time.
This is the elusive gravitational radiation, launched far away in the cosmos by mass in cataclysmic motion, that my Georgia Tech colleagues Laura Cadonati and Deirdre Shoemaker and the LIGO Collaboration seem very likely to have observed directly for the very first time, through a textbook interplay between deeply challenging experiment and cutting-edge computation. Today’s sensation is tomorrow’s calibration, and many physicists are expecting that, before long, we shall be hearing exciting new messages about the cosmos, whispered to us in the language of light’s gravitational cousin.”
VIDEO:
Chirp pattern of gravitational waves detected by LIGO on September 14, 2015
Information provided by Georgia Institute of Technology